Muscle Fatigue During Resistance Training: Mechanisms and Implications
- Kaveshan Naidoo
- Jul 1, 2024
- 5 min read
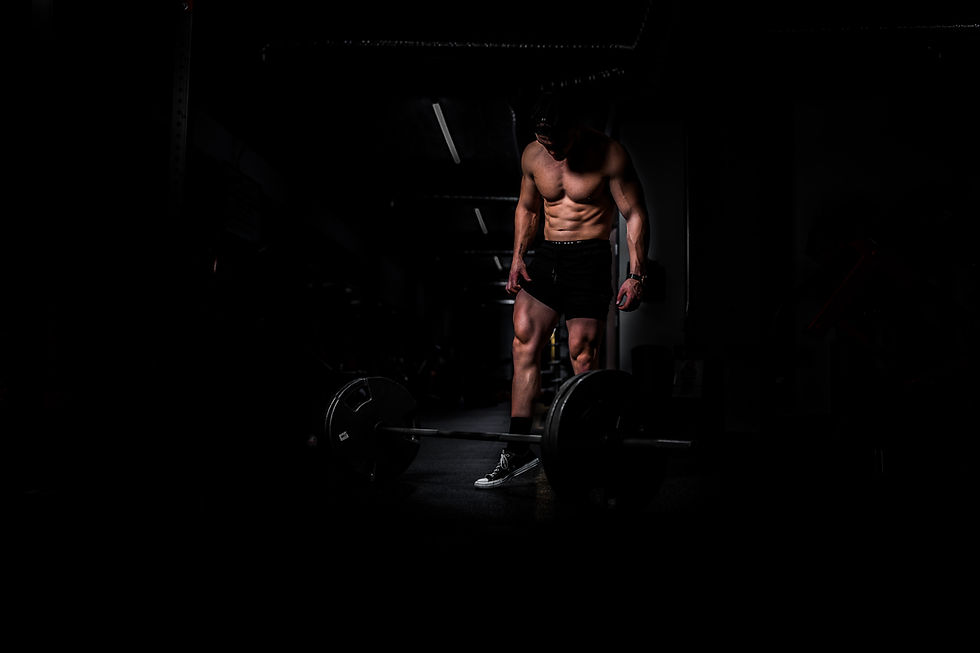
As a strength athlete or bodybuilder, you've likely experienced that burning sensation and loss of force production as you grind through those final tough reps of a set. This phenomenon, known as muscle fatigue, plays a crucial role in the adaptations we seek from resistance training. But what exactly is happening in our muscles and nervous system as fatigue sets in? And how can we leverage our understanding of fatigue to optimize our training? Let's dive into the fascinating science behind muscle fatigue during resistance exercise.
The Neuromuscular Basis of Fatigue
At its core, muscle fatigue refers to the exercise-induced decline in our ability to produce force [1]. As we perform repetitive muscle contractions during a set, several physiological changes occur that contribute to fatigue:
1. Depletion of energy substrates: Our muscles rely on ATP (adenosine triphosphate) for energy. As we exercise, ATP stores become depleted faster than they can be replenished [2].
2. Accumulation of metabolites: Byproducts of muscle contraction like hydrogen ions and inorganic phosphate build up, interfering with the muscle's contractile processes [2].
3. Altered calcium handling: Calcium plays a vital role in muscle contraction. Fatigue can disrupt the release and uptake of calcium within muscle fibers [2].
4. Central nervous system fatigue: The brain and spinal cord may reduce neural drive to the muscles as a protective mechanism [1].
Interestingly, research has shown that different types of fatigue manifest depending on the intensity and duration of exercise. For example, high-intensity, short-duration efforts like a 1-rep max attempt primarily induce central fatigue, while moderate-intensity, longer-duration sets lead to more peripheral fatigue within the muscle fibers themselves [3].
Monitoring Fatigue During Resistance Exercise
Scientists use several methods to assess neuromuscular fatigue during resistance training:
1. Force production: Measuring the decline in maximum voluntary contraction (MVC) force is a direct way to quantify fatigue [1].
2. Electromyography (EMG): This technique measures electrical activity in muscles. As fatigue sets in, characteristic changes in the EMG signal occur, such as a decrease in the median frequency [4].
3. Mechanomyography (MMG): Similar to EMG, but measuring mechanical oscillations of muscle fibers [5].
4. Ratings of perceived exertion (RPE): While subjective, RPE can provide valuable insight into an individual's fatigue state [6].
A study by Keller et al. (2018) used these techniques to examine fatigue during a sustained, submaximal leg extension held at a constant perceived effort (RPE = 5 out of 10). They found that participants had to progressively reduce force output to maintain the same RPE, with quadratic decreases in force production over time. Interestingly, mechanomyography amplitude increased throughout the task, suggesting altered motor unit recruitment patterns as fatigue developed [6].
Motor Unit Behavior and Fatigue
Motor units, consisting of a motor neuron and the muscle fibers it innervates, are the fundamental functional units of our neuromuscular system. As fatigue develops during resistance exercise, fascinating changes occur in motor unit behavior:
1. Increased recruitment: To maintain force output as fatigue sets in, our nervous system recruits additional motor units, particularly larger, higher-threshold units [7].
2. Altered firing rates: Motor unit firing rates may initially increase to compensate for fatigue, but eventually decrease as exhaustion approaches [7].
3. Synchronization: Fatigued muscles show increased motor unit synchronization, potentially as a compensatory mechanism [8].
A recent study by Nishikawa et al. (2024) used advanced EMG techniques to examine motor unit behavior before and after a challenging set of leg extensions. They found that motor units with higher recruitment thresholds showed greater fatigue-induced changes in firing rates compared to lower-threshold units. This suggests that the high-threshold motor units associated with type II muscle fibers may be more susceptible to fatigue during resistance training [9].
Practical Implications for Resistance Training
Understanding the mechanisms of muscle fatigue has important implications for designing effective resistance training programs:
1. Progressive overload: By strategically inducing and managing fatigue, we can create the stimulus needed for muscular adaptations. Gradually increasing volume, intensity, or frequency over time ensures continued progress [10].
2. Rest periods: Shorter rest periods between sets lead to greater accumulated fatigue, potentially beneficial for hypertrophy. Longer rests allow for more complete recovery, favoring strength development [11].
3. Exercise selection and order: Compound exercises typically induce more systemic fatigue than isolation movements. Strategically ordering exercises can optimize the fatigue stimulus [12].
4. Training to failure: While training to momentary muscular failure induces high levels of fatigue and can be effective for hypertrophy, it may not be necessary for all sets and can increase recovery demands [13].
5. Periodization: Properly managing fatigue through periodized training plans helps prevent overtraining and optimizes long-term progress [14].
Conclusion
Muscle fatigue is a complex phenomenon involving multiple physiological systems. By understanding the mechanisms behind fatigue during resistance training, we can make more informed decisions about program design and exercise execution. While fatigue is often seen as a negative, when properly managed, it serves as a powerful stimulus for the adaptations we seek in the weight room. So embrace the burn, but do so intelligently!
References:
1. Enoka, R. M., & Duchateau, J. (2008). Muscle fatigue: what, why and how it influences muscle function. The Journal of physiology, 586(1), 11-23.
2. Allen, D. G., Lamb, G. D., & Westerblad, H. (2008). Skeletal muscle fatigue: cellular mechanisms. Physiological reviews, 88(1), 287-332.
3. Taylor, J. L., & Gandevia, S. C. (2008). A comparison of central aspects of fatigue in submaximal and maximal voluntary contractions. Journal of Applied Physiology, 104(2), 542-550.
4. González-Izal, M., Malanda, A., Gorostiaga, E., & Izquierdo, M. (2012). Electromyographic models to assess muscle fatigue. Journal of electromyography and kinesiology, 22(4), 501-512.
5. Hill, E. C., Housh, T. J., Smith, C. M., Schmidt, R. J., & Johnson, G. O. (2018). Mechanomyographic amplitude and mean power frequency versus torque relationships during isokinetic and isometric muscle actions of the biceps brachii. Journal of electromyography and kinesiology, 41, 36-41.
6. Keller, J. L., Housh, T. J., Hill, E. C., Smith, C. M., Schmidt, R. J., & Johnson, G. O. (2018). Neuromuscular responses of recreationally active women during a sustained, submaximal isometric leg extension muscle action at a constant perception of effort. European journal of applied physiology, 118(12), 2499-2508.
7. Contessa, P., De Luca, C. J., & Kline, J. C. (2016). The compensatory interaction between motor unit firing behavior and muscle force during fatigue. Journal of neurophysiology, 116(4), 1579-1585.
8. Boonstra, T. W., Daffertshofer, A., van Ditshuizen, J. C., van den Heuvel, M. R., Hofman, C., Willigenburg, N. W., & Beek, P. J. (2008). Fatigue-related changes in motor-unit synchronization of quadriceps muscles within and across legs. Journal of electromyography and kinesiology, 18(5), 717-731.
9. Nishikawa, T., Hirono, T., Holobar, A., Kunugi, S., Okudaira, M., Ohya, T., & Watanabe, K. (2024). Acute effects of caffeine or quercetin ingestion on motor unit firing pattern before and after resistance exercise. European Journal of Applied Physiology, 124(6), 1645-1658.
10. Schoenfeld, B. J., Grgic, J., Ogborn, D., & Krieger, J. W. (2017). Strength and hypertrophy adaptations between low-vs. high-load resistance training: a systematic review and meta-analysis. The Journal of Strength & Conditioning Research, 31(12), 3508-3523.
11. Grgic, J., Schoenfeld, B. J., Skrepnik, M., Davies, T. B., & Mikulic, P. (2018). Effects of rest interval duration in resistance training on measures of muscular strength: a systematic review. Sports Medicine, 48(1), 137-151.
12. Simão, R., de Salles, B. F., Figueiredo, T., Dias, I., & Willardson, J. M. (2012). Exercise order in resistance training. Sports medicine, 42(3), 251-265.
13. Davies, T., Orr, R., Halaki, M., & Hackett, D. (2016). Effect of training leading to repetition failure on muscular strength: a systematic review and meta-analysis. Sports Medicine, 46(4), 487-502.
14. Lorenz, D. S., Reiman, M. P., & Walker, J. C. (2010). Periodization: current review and suggested implementation for athletic rehabilitation. Sports health, 2(6), 509-518.
Comentarii